Superconducting Nanowire Single-Photon Detectors (SNSPDs) are highly sensitive devices used to detect single photons with exceptional timing precision and efficiency. SNSPDs have applications in quantum optics, secure communications, deep-space optical communication, and biomedical imaging due to their low noise, fast response, and near-unity detection efficiency.
SNSPDs operate on the principle of superconductivity, where a thin nanowire is cooled below its critical temperature, allowing it to enter a superconducting state with zero electrical resistance. When a photon strikes the nanowire, it disrupts the superconductivity locally, creating a resistive hotspot. This transition generates a measurable voltage pulse, indicating the arrival of a single photon.
The SNSPD consists of a thin (≈ 5 nm) and narrow (≈ 100 nm) superconducting nanowire. The length is typically hundreds of micrometers, and the nanowire is patterned in a compact meander geometry to create a square or circular pixel with high detection efficiency. The nanowire is cooled well below its superconducting critical temperature and biased with a DC current that is close to but less than the superconducting critical current of the nanowire. A photon incident on the nanowire breaks Cooper pairs and reduces the local critical current below that of the bias current.
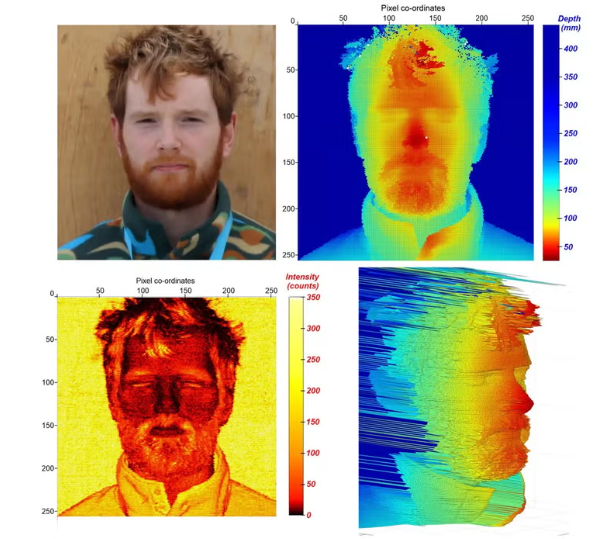
A new study—led by Heriot-Watt University in Edinburgh and including scientists from NASA’s Jet Propulsion Laboratory (JPL), MIT, and the University of Glasgow—details the creation of a superconducting LiDAR system capable of accurately capturing 3D data up to an astonishing one kilometer away, with resolution at the millimeter-scale.
Research University in Edinburgh | Heriot-Watt University
This results in the formation of a localized non-superconducting region, or hotspot, with finite electrical resistance. This resistance is typically larger than the 50 ohm input impedance of the readout amplifier, and hence most of the bias current is shunted to the amplifier. This produces a measurable voltage pulse that is approximately equal to the bias current multiplied by 50 ohms. With most of the bias current flowing through the amplifier, the non-superconducting region cools and returns to the superconducting state. The time for the current to return to the nanowire is typically set by the inductive time constant of the nanowire, equal to the kinetic inductance of the nanowire divided by the impedance of the readout circuit.
Proper self-resetting of the device requires that this inductive time constant be slower than the intrinsic cooling time of the nanowire hotspot.
While the SNSPD does not match the intrinsic energy or photon-number resolution of the superconducting transition edge sensor, the SNSPD is significantly faster than conventional transition edge sensors and operates at higher temperatures. A degree of photon-number resolution can be achieved in SNSPD arrays, through time-binning[21] or advanced readout schemes. Most SNSPDs are made of sputtered niobium nitride (NbN), which offers a relatively high superconducting critical temperature (≈ 10 K) which enables SNSPD operation in the temperature range 1 K to 4 K (compatible with liquid helium or modern closed-cycle cryocoolers). The intrinsic thermal time constants of NbN are short, giving very fast cooling time after photon absorption (<100 picoseconds).
Key Components
Superconducting Nanowire
Typically made from materials such as niobium nitride (NbN) or tungsten silicide (WSi).
Nanowire thickness ranges from a few nanometers to tens of nanometers.
Cryogenic Cooling System
SNSPDs require cooling to temperatures below 2.5 K, typically achieved using a cryostat.
Cooling minimizes thermal noise and maintains superconductivity.
Optical Coupling System
Optical fibers or lenses direct photons to the nanowire for efficient detection.
Readout Electronics
Low-noise amplifiers and fast signal processing units analyze the generated voltage pulses.
Performance Metrics
Detection Efficiency
High-efficiency SNSPDs can achieve detection probabilities exceeding 90%.
Timing Resolution
SNSPDs provide picosecond-level timing precision, crucial for quantum communication and time-correlated single-photon counting.
Dark Count Rate
The rate of false detection events is extremely low, enhancing signal integrity.
Jitter
The timing uncertainty in photon detection is minimized, improving precision in time-sensitive applications.
Applications
Quantum Cryptography
SNSPDs enable secure quantum key distribution (QKD) by detecting entangled photons with high accuracy.
Astronomy and Space Communication
Used in deep-space optical communication systems to detect weak photon signals from distant sources.
Biomedical Imaging
Applied in fluorescence lifetime imaging microscopy (FLIM) and positron emission tomography (PET).
LIDAR and Remote Sensing
Enhances high-resolution depth mapping for autonomous vehicles and geospatial applications.
Challenges and Future Prospects
Cryogenic Requirements: The necessity of ultra-low temperatures limits portability and increases operational complexity.