BCI technology allows a human brain and an external device to talk to one another—to exchange signals. It gives humans the ability to directly control machines, without the physical constraints of the body.
A brain–computer interface (BCI), sometimes called a neural control interface (NCI), mind–machine interface (MMI), direct neural interface (DNI), or brain–machine interface (BMI), is a direct communication pathway between an enhanced or wired brain and an external device. BCIs are often directed at researching, mapping, assisting, augmenting, or repairing human cognitive or sensory-motor functions.
Brain-computer interfaces (BCIs) acquire brain signals, analyze them, and translate them into commands that are relayed to output devices that carry out desired actions. BCIs do not use normal neuromuscular output pathways.
The main goal of BCI is to replace or restore useful function to people disabled by neuromuscular disorders such as amyotrophic lateral sclerosis, cerebral palsy, stroke, or spinal cord injury. From initial demonstrations of electroencephalography-based spelling and single-neuron-based device control, researchers have gone on to use electroencephalographic, intracortical, electrocorticographic, and other brain signals for increasingly complex control of cursors, robotic arms, prostheses, wheelchairs, and other devices.
EEG nodes + frequency/amplitude modulated wire loops, designed to
produce magnetic fields of modulated field intensity and size (I assume
everyone reading this is familiar with transcranial magnetic
stimulation). Due to the mathematical behavior of this, we can utilize
the formulas involving Columb and Lorenz forces to predict the spatial
distribution spikes in energy that form bands in the magnetic field
produced. Utilizing these principles, we can then take advantage of the
principles of superposition and cancellation when multiple such
modulated fields are produced to increase or decrease the intense the
charge passing through the neurons in the regions of overlap.
Similarly, we are also able to predict the skew such signals will cause
in nearby EEG nodes, also in fixed spatial positions. This would allow
for fine resolution activation of groups of neurons, as well as
suppression of existing neural firing via interactions between membrane
polarization and intersecting ‘troughs’ in the wave patterns’ spatial
positions.
This is merely a refinement of the low resolution proof of concept
performed by Stocco and Rao (2013), and later replicated by others at
the University of Washington.
Due to the necessity of fixed spatial position for reliably calculating
overlapping superposition and cancellation of the electromagnetic fields
within the brain (linked to the particular effects of cellular
structure on the field produced by it’s cell body polarization),
intracranial implantation of EEG and magnetic induction loops would be
ideal. However the shifting position of the brain within the skull would
ultimately necessitate the use of algorithms interpreting the shape of
the field as perceived by EEG nodes regardless, in order to have a
meaningful map on which to project changes via magnetic stimulation.
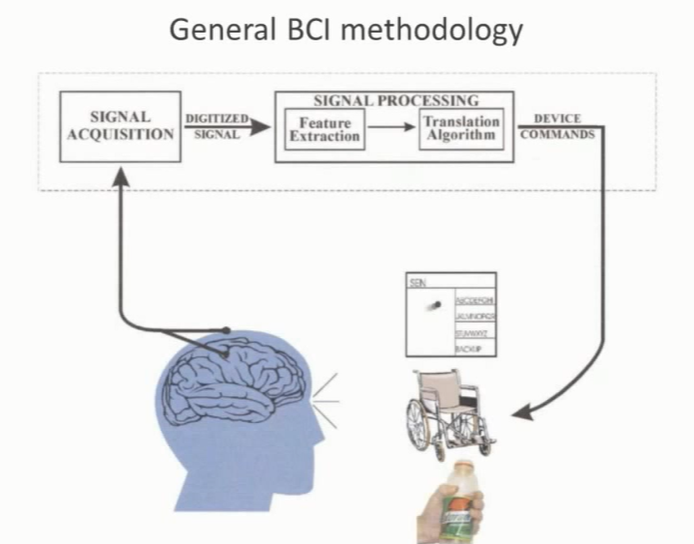
This technique would allow for a high amount of resolution with limited
stimulation sources of relatively lower individual intensity, with
exponential gain in resolution and for each interacting magnetic
stimulation node influencing a region. From this point, it becomes a
matter of software, rather than hardware.
Similarly this relationship between cell membrane polarization, combined
with spikes from potentiation, upon the EMF (electromagnetic field) of
the individual neurons is also, by its nature, a product of these
patters of calculable superposition and cancellation. Operating from
this model, the intensity in the fields perceived via EEG must also,
according to our current understanding of the EMF fields involved, carry
calculable information regarding the base polarization, and specific
firing activity, that may be mathematically reduced via the spatial
positions of cancellation and superposition, and their electromagnetic
intensity.
Brain-computer interfaces may also prove useful for rehabilitation after stroke and for other disorders. In the future, they might augment the performance of surgeons or other medical professionals. Brain-computer interface technology is the focus of a rapidly growing research and development enterprise that is greatly exciting scientists, engineers, clinicians, and the public in general. Its future achievements will depend on advances in 3 crucial areas. Brain-computer interfaces need signal-acquisition hardware that is convenient, portable, safe, and able to function in all environments. Brain-computer interface systems need to be validated in long-term studies of real-world use by people with severe disabilities, and effective and viable models for their widespread dissemination must be implemented. Finally, the day-to-day and moment-to-moment reliability of BCI performance must be improved so that it approaches the reliability of natural muscle-based function.
Related Articles on BCI –
https://www.brown.edu/news/2021-03-31/braingate-wireless
http://www.brainvision.co.uk/blog/2014/04/the-brief-history-of-brain-computer-interfaces/