Terahertz spectroscopy detects and controls properties of matter with electromagnetic fields that are in the frequency range between a few hundred gigahertz and several terahertz (abbreviated as THz).
Terahertz (THz) represents the portion of the electromagnetic radiation between the microwave and the infrared region.
Terahertz (THz) spectroscopy systems are widely employed to retrieve the chemical and material composition of a sample. This is single-handedly the most important driving motivation in the field and has largely contributed to shaping THz science as an independent subject.
It is within the frequency range of 0.1–10 THz, corresponding to wavelengths of radiation from 3000 to 30 µm. Terahertz radiation is also known as terahertz gap, terahertz waves, T‐waves, terahertz light, T‐light, or T‐lux. This form of electromagnetic radiation is less known, due to the limited access to technology for generating and detecting radiation [1]. There are a number of reviews on the different technologies for generating and detecting terahertz .
Terahertz waves are nonionizing, noninvasive, and penetrable to many materials with a depth of penetration lower than that of microwave radiation. Terahertz radiation also tends to be very sensitive to various kinds of resonances such as vibrational, translational, rotational, torsional, and conformational states, enabling it to provide information on molecules that are inaccessible with other analytical and imaging techniques. These unique characteristics make them suitable for identifying, analyzing, or imaging a variety of materials.
The limited availability of high-resolution imaging devices, however, still represents a major technological challenge in this promising field of research. In this theoretical work, we tackle this challenge by developing a novel nonlinear ghost imaging approach that conceptually outperforms established single-pixel imaging protocols at inaccessible wavelengths. Our methodology combines nonlinear THz generation with time-resolved field measurements, as enabled by state-of-the-art time domain spectroscopy techniques.
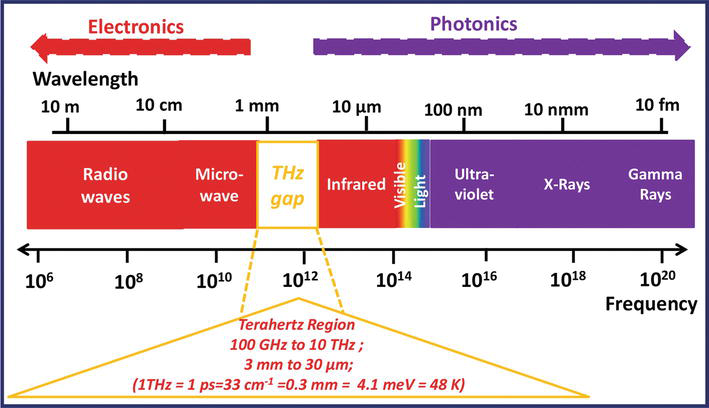
As an ideal application target, it considers hyperspectral THz imaging of semitransparent samples with nonnegligible delay contribution, and demonstrate how time-resolved, full-wave acquisition enables accurate spatiotemporal reconstruction of complex inhomogeneous samples.
Unlike Raman and Infrared spectroscopy, the development of techniques for generating, manipulating, and detecting terahertz radiation is still in its early stages. Although terahertz radiation was deemed suitable for imaging and other applications, technologies to harness such capabilities were practically nonexistent.
On one hand, high terahertz frequencies do not lend themselves to be estimated with electronic counters employed in the detection of optical waves; instead various properties of wavelength and energy are harnessed to characterize terahertz radiation. On the other hand, at such higher frequencies electronic devices utilized in generation and manipulation of radio waves and microwaves become less efficient.
Thus with the exception of the terahertz gap, technology for the generation, manipulation, and everyday application of a vast majority of the electromagnetic spectrum has been developed. Significant progress made in terahertz technology has led to an increase in the different types of terahertz instrumentation available on the market with application for diverse fields.
Security screening
Security screening is one of the most common applications of terahertz technology. Terahertz does not only give information about the presence of concealed items but also has the capacity to identify the composition of the materials in question. In recent years numerous investigations have been conducted on the capability of terahertz to detect explosives at airports and other sensitive places .
The advantage of terahertz radiation over X‐ray, also used for security screening, is its low energy and nonionizing nature. The ionizing nature of X‐rays makes the regular exposure of it harmful to people. The unique ability of Terahertz waves to penetrate a wide range of packaging materials makes it possible to detect weapons, explosives, and potentially explosive devices concealed within these materials.
Medical applications of terahertz technology
The last couple of decades have seen the development of new terahertz techniques for imaging and sensing purposes. Like X‐ray, terahertz radiation is capable of generating two‐dimensional images of different kinds of objects owing to the fact that terahertz waves travel through certain materials such as semiconductors and plastics but are not transparent to others such as metallic and polarizing materials. Terahertz waves are preferable over X‐rays for medical imaging since they are nonionizing and to a large degree harmless to the recipient. Terahertz waves are currently under investigation for use as an imaging modality to visualize cancerous tissues. According to the American Cancer Society, cancer is the second leading cause of death in the U.S., second only to heart disease, and accounts for nearly one in every four deaths. Early detection could mean the difference between life and death for many patients but there is a shortfall in the current imaging techniques. New terahertz spectroscopic studies on cancer could potentially provide novel techniques for the early detection of the disease.
In fact, there has been a steady increase in the number of studies demonstrating the potential use of THz radiation for the imaging and detection of skin tissues and cancerous cells [20, 38–40]. Since terahertz radiation is readily absorbed by water and other polar liquids, the method lends itself well to imaging most organic tissue. Water has intense absorption in the terahertz portion of the electromagnetic spectrum and with the concentration of water in cancerous cells significantly different from that in healthy cells, the variation in the water absorption of terahertz radiation becomes the basis of differentiation of normal cells from cancerous cells. Using THz pulse imaging, Woodward et al. were able to differentiate basal cell carcinoma, a type of skin cancer, from healthy tissues. There have also been studies on the THz pulse imaging in breast cancer.
A great number of biomolecules including DNA/RNA, carbohydrates, amino acids/peptides, proteins, cells, and tissues have been investigated using terahertz radiation. The low‐frequency internal helical vibration of DNA involving hydrogen bond of DNA base pairs is reflected in the terahertz frequency absorption spectra. Such sensitivity of biomolecules to terahertz radiation has been utilized for the characterization of biological materials. Globus et al. used terahertz spectroscopy to probe DNA polymers in which they were able to prove the dependence of the mode strength on the polarization of the terahertz field with respect to the molecule alignment and thereby concluded that theoretical modeling, combined with measured data, may be used to directly assign vibrational modes to specific structural features of the macromolecule. Using transmission time‐domain terahertz spectroscopy and continuous wave terahertz imaging, Wahaia et al. were able to distinguish normal cells from cancerous cells and were, consequently, able to detect colon cancer.
Quantitative detection of impurities in organic crystals –
Quantitative detection of impurities in organic crystals was demonstrated by accurately measuring absorption frequencies using a continuous wave gallium phosphide terahertz spectrometer. THz spectra of l-asparagine monohydrate doped with l-aspartic acid at 0.05 to 12.5 wt % were obtained at 10 K. The three lowest frequency absorption peaks were baseline-resolved, allowing them to be examined independently. Using a least-squares curve fitting technique, impurities were detected at levels as low as 500 ppm. The sensitivity and detection limits of the technique depended strongly on the nature of both the host and the impurities.